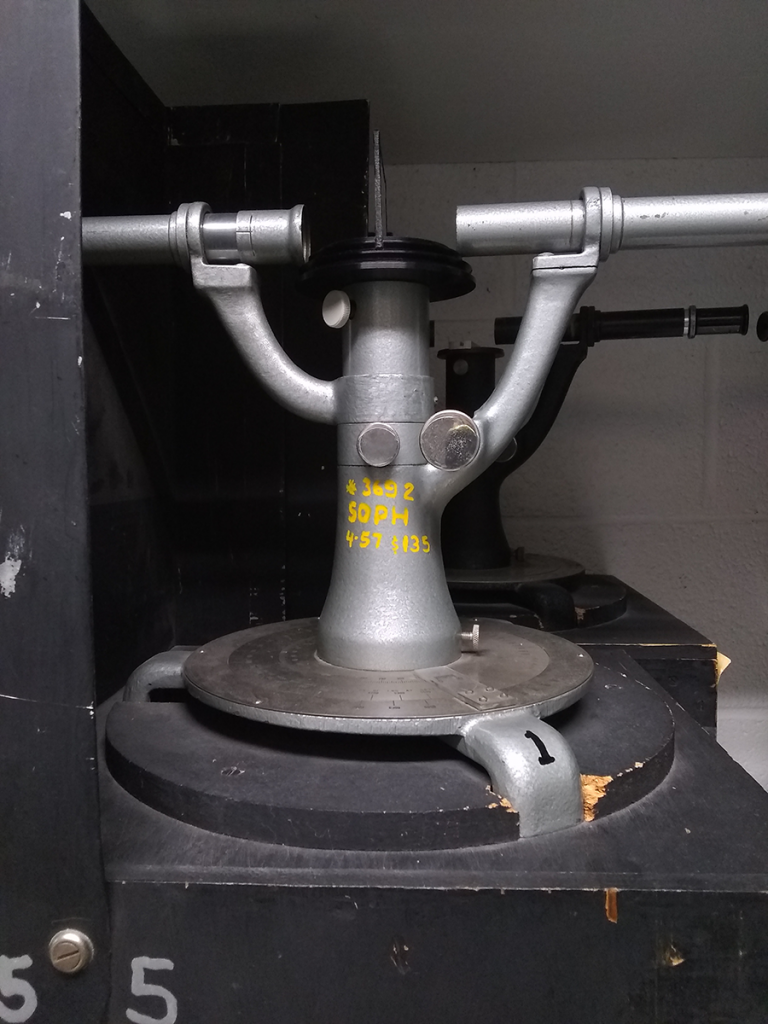
Sometimes, we have old equipment which is rarely, if ever used. Case in point: the mid-20th-century spectroscopes which have been supplanted by digital spectrometers. They’re both effective tools for examining a spectrum of light, one by eye and the other fed by a USB cable. Using a diffraction grating, they split light into its constituent spectrum – its rainbow, more or less – and can identify the presence of individual wavelengths. Not something our eyes can do, as they blend everything together, though that’s very helpful in most situations, such as reading this on your screen.
Summing bands of reddish, greenish, and bluish into a broad rainbow of colors is one neat-o trick.
With a diffraction grating, reflection grating, or prism, you can refract light out along a range of angles which correspond to its constituent wavelengths. Put a sensor at a known angle – your eye or a semiconductor exhibiting the photoelectric effect – and you know the wavelength if you sense a photon. It’s a simple piece of information which can be used to unlock a staggering amount of interesting, related information about what you’re observing.
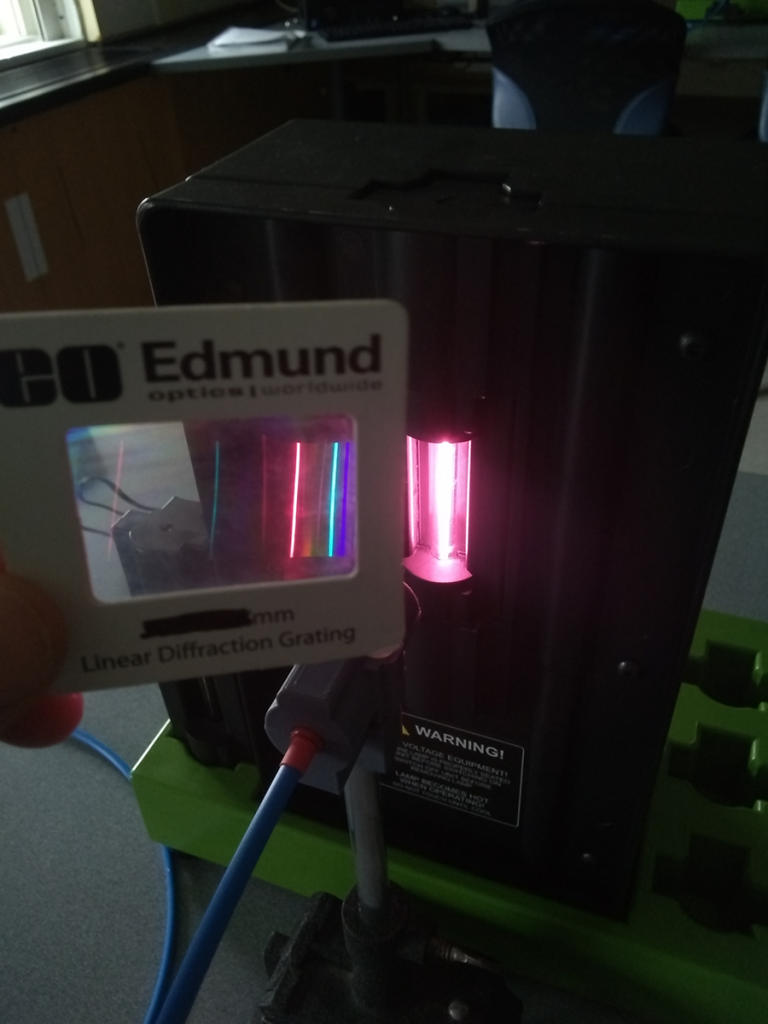
You can also use a diffraction grating to get a quick sense of the entire visible spectrum of a source by holding it off to the side. Remember: the angle of the light’s path change as it refracts, so you’re trying to angle it back to your eye. Hydrogen has a distinctly pinkish-purplish hue when excited at high voltage, and you can see the dominant red and blue lines in its spectrum. With just that one electron to absorb energy and emit photons, the spectrum can only be so complicated.
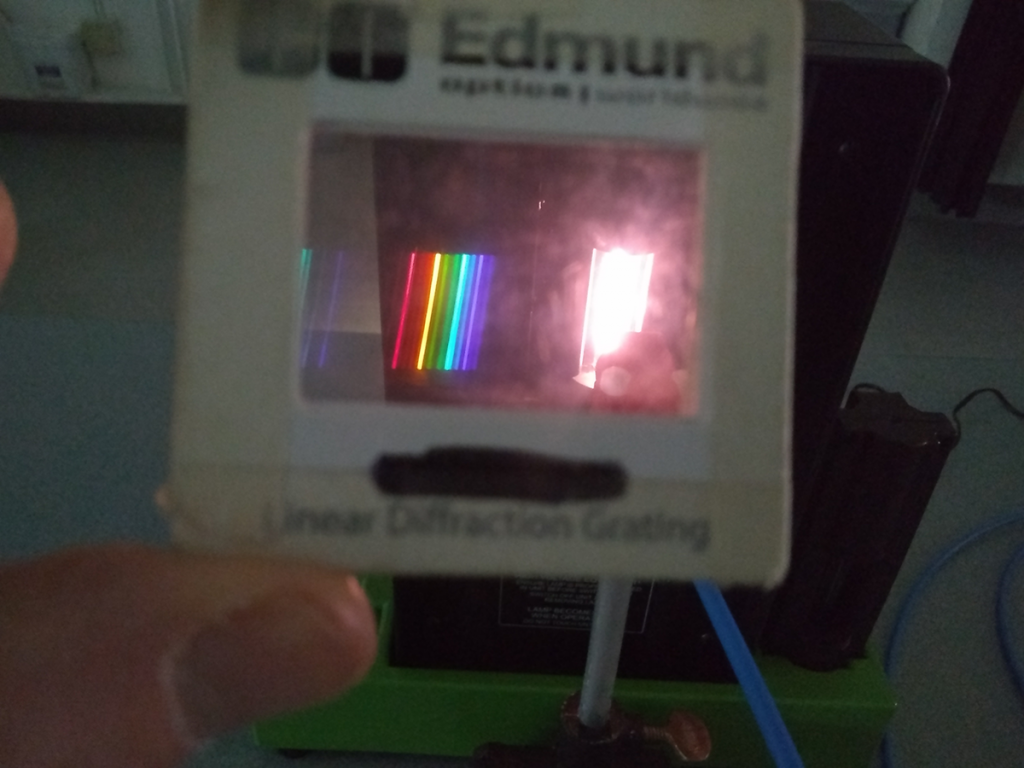
That’s in contrast to helium, with its two electrons. The spectrum doesn’t look white, per se, but is much more filled out than hydrogen. Look at those spectral lines, and there are so many more! They’re distinct, measurable, and provide a “fingerprint” that can be immensely useful for scientific study. Or for just looking cool.