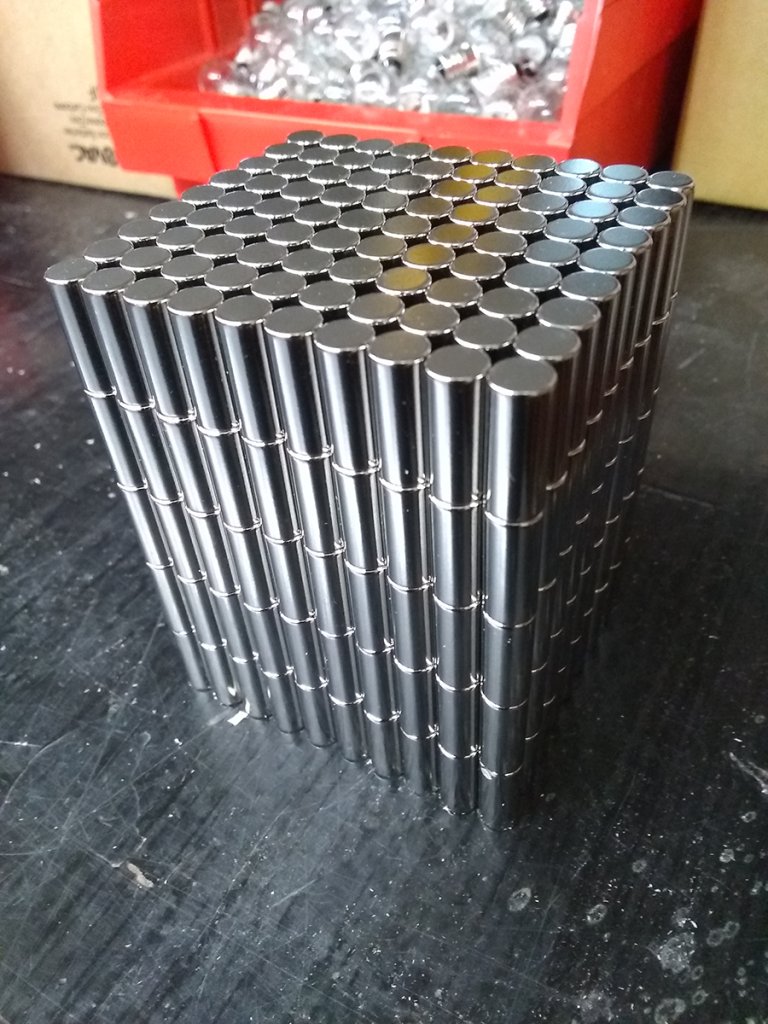
‘Tis that most joyous of days in the beginning of the semester: physics toy kit day! A bag full of odds and ends, perfect for playing, experimenting, and providing tactile bits to use when working through physics problems. Batteries, compasses, various wires, polarizing filters, nails, magnets, and balloons. Always balloons.
Each kit contains two small neodymium magnets, because magnets are amazing. First, you’re bound to stick them together, then spin one around and feel them repel. Surprisingly strong such wee little cylinders. Then check what they stick to around you: whether or not they feel attracted to stainless steel is always intriguing. (The answer is: depends on the type of steel and how it was formed.) Stick them together across a string and let it hang: you’ve built a compass!
Pay attention to the time and location of the sun – or Polaris if you’re pulling an all-nighter – and you can tell which pole of your magnets is which. Maybe it’ll come in handy?