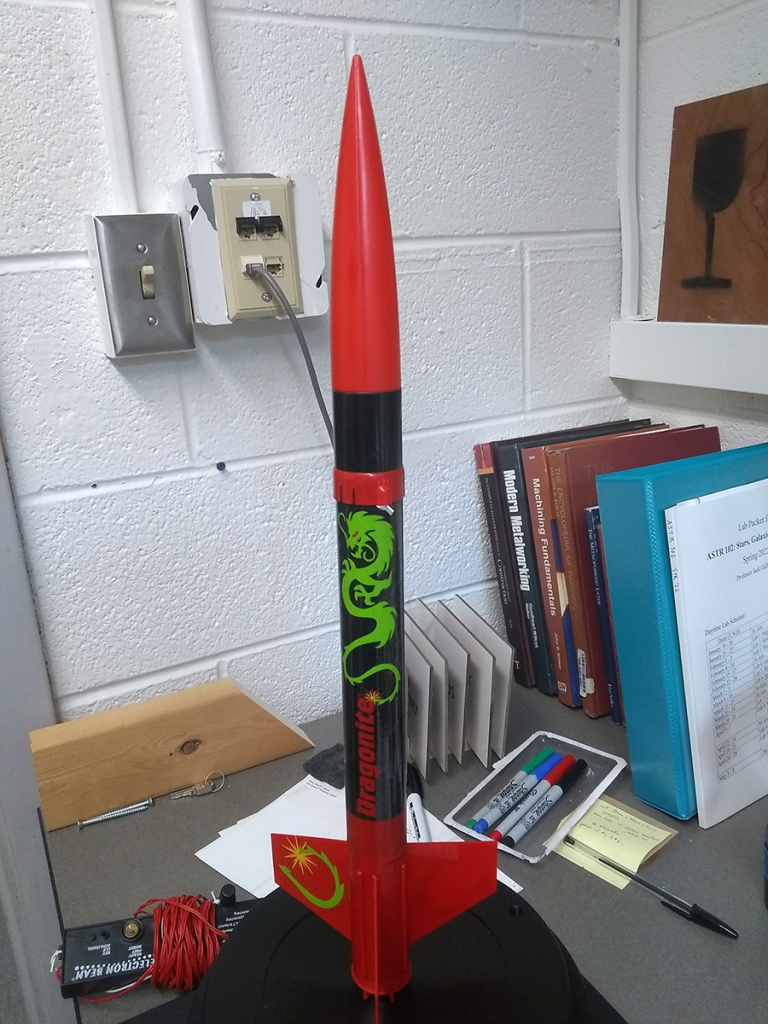
It’s nearly rocket day! Okay, well, it’s nearly time for a physics lecture on momentum conservation – good ol’ Newtonian mechanics – and nothing livens up a discussion of theory and mathematical modeling like making stuff shoot up into the sky. Or, quite possibly, fail to shoot up into the sky, but we’ve been running some advance tests and prepping backup plans because we really, really want things to go zoom.
Zoom, not boom. It’s much more of a hissing zzziiippp than anything else.
The demonstration usually shows three different rockets in succession, each more impressive than the last. The first one, a soda-bottle water rocket, actually illustrates the principle best. Pressurized water shoots out and down, so the rocket moves up. Mass, velocity, terrible aerodynamics. Occasional light spray, so keep your distance.
Then it’s off to model rocket land, with high-velocity solid fuel instead of water. Less mass but at a much, much higher velocity, and in no time that B-size engine has launched the little cardboard-and-plastic rocket high enough to be a speck that’s hard to discern. As long as the weather isn’t terrible, though, a standard B launch is not only recoverable, but entirely possible to catch before it reaches the ground, drifting lazily beneath its parachute.
Scaling on up to a C-size engine, we’ll have our final launch. Bigger engine, more momentum, and even the slightest breeze ensures it’ll drift far beyond our sight and ability to track. Anyone so lucky as to find the rocket afterward can keep it.
If it’s you, maybe stop on by and let us know?