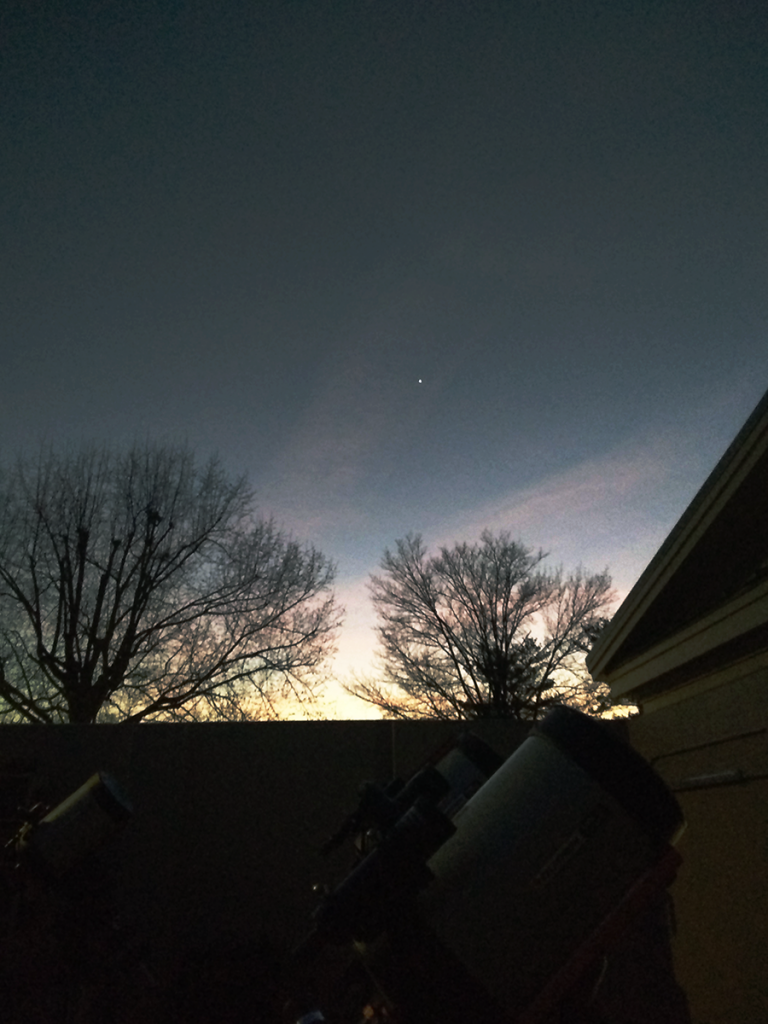
Before the night stars appear, there’s Venus setting in the west. Holy cow, is she ever bright.
Discoveries in the Physics & Astronomy shop | Science, curiosities, and surprises
Before the night stars appear, there’s Venus setting in the west. Holy cow, is she ever bright.
In our cabinet of meteorites – because everyone has one of those in an odd corner, right? – we have a number of surprisingly-dense space rocks. You can’t really hurt them, considering they’ve already been dropped far harder than any puny human can manage, so occasionally we get to pass them around.
Here we have the Plainview meteorite, from Hale County, Texas. Discovered in 1917, the original find weighed over 400 kg (a lot!), and our little chunk is a mere 184 g. Isn’t it adorable?
Our old iron optics rails get very little use anymore, as we phase them and their accessories out. Most of them, that is.
We may not use the old glass lenses much – sometimes, not often – but the spring-loaded holders still come out from time to time. They grip certain oddly-shaped objects well, and their heavy iron bases do an excellent job of keeping things like fiber optic cables upright and in place.
Rapidly approaching 60 years old, lens holder. April 1963, $6.25. That’s $61.31 in today’s dollars.
It is possible to snap a photo of the moon through the antique Clark refractor telescope. It’s just not very easy.
For the most part, deciding on constellations is hard. A few really stand out (Orion, Cassiopeia) as do a number of bold asterisms (the Big Dipper in Ursa Major, the Teapot in Sagittarius). The rest of the sky, where there are stars but no super-obvious pattern stands out? Oof.
Picking all 88 sounds like a beastly challenge.
Still… sometimes it seems like someone just starting phoning it in in the end.
Declaring Norma to be a carpenter’s square isn’t helping your case.
There’s a funny thing about important scientific discoveries. The effort and time and careful data collection and building atop previous understandings and innovations and everything else is daunting, difficult, and a massive undertaking. Critical details and a fine understanding may take months, years, or entire careers. A general grasp, though?
Sometimes, you can explain the gist of things with stuff that’s just lying around.
Hubble’s Law, also known as the Hubble-Lemaître Law, describes the expansion of the universe. Galaxies are moving away from ours, and the further away they are, the faster they’re moving. Getting there relied on the Friedmann equations – themselves built upon Einstein’s general relativity – plus Slipher’s redshift measurements of distant galaxies, plus the debates between Shapley and Curtis, plus an understanding of the relationship between luminosity and period in the pulsations of Cepheid variable stars. (They’re like the drinking bird toys of stars.) Plus more, and more, but you get it. A lot goes into explaining the expansion of the universe when all you’ve got is a telescope and spectrometer.
Hubble ran into a real hiccup here. If everything in the universe is moving away from us, and we can correlate the distance and speed in any direction, doesn’t that imply that we’re at the center of the universe? Turns out, no. We’re not.
And you can illustrate the principle with a Slinky, a ruler, and some paper clips.
We get a lot of mileage out of the Slinky.
When astronomers study objects they can’t reach, they’re typically limited to visual clues to glean information. Sometimes that’s color variation, like when the ejecta from a crater redistribute layers of rock and soil. Laid down at different times, and made of different materials, the dark and light rings and patches can provide a great deal of insight into how and when a lunar crater formed, for example.
The moon is somewhat less vividly colored than a sink full of tempera paint powder, of course. Electroshock hues make the distinctions easier for the students. We have black, brown, and white in the mix. They work just as well, but never elicit the excited reaction of a brilliant orange or a neon-level magenta.
Intended for mixing your own paint, these are effectively the same as the bright, thick paints in nearly every kids’ art classroom you’ve ever seen. Combined with the play sand, the whole lab starts to smell a little bit like a fun day at preschool.
In the end, it all becomes a smeared, brownish-gray mix of sand, pigment, and the occasional lost marble or ball bearing. That and a room where every horizontal surface has a new layer of fine, fine dust…
You know what makes great examples of craters in a lab setting? Play sand. Powdered tempera paint. Slingshots. A variety of round projectiles.
It’s going to be a spectacular mess next week.
The moon enters totality, as viewed from the SkyCam, 4:55 to 5:20 am. Not nearly as cool as in person, but eclipses are always cool.
Meteorites – those shooting stars which don’t completely burn up entering our atmosphere and then crash to the ground – can be made of all sorts of stuff. The most commonly found in museums and collections are metallic, not because they’re the most frequent type of meteorite, but because they’re the most likely both to survive entry/impact and to be discovered. A stony meteorite might look remarkably like an ordinary rock. A big chunk of warped iron just sitting on the ground? Slightly more conspicuous.
We have a few meteorites and pieces of meteorites on display, including this big slab. Cut, polished, and given an acid treatment, it shows off its internal crystalline structure. Primarily iron and nickel in two different crystalline shapes, it has a characteristic pattern known as a Widmanstätten pattern. Given a sufficiently long cooling period to enable crystal formation – typically on the order of millions of years – it produces this distinct appearance that highlights its extraterrestrial provenance.
Can’t do this stuff in a lab is what we’re saying.
The acid-etching process enhances the pattern where the high-nickel taenite alloy is more resistant to the acid than the low-nickel kamacite, turning a smooth, polished surface into one that looks, well, really cool.